- In-Stock Tumor Cell Lines
- Human Orbital Fibroblasts
- Human Microglia
- Human Pulmonary Alveolar Epithelial Cells
- Human Colonic Fibroblasts
- Human Type II Alveolar Epithelial Cells
- Human Valvular Interstitial Cells
- Human Thyroid Epithelial Cells
- C57BL/6 Mouse Dermal Fibroblasts
- Human Alveolar Macrophages
- Human Dermal Fibroblasts, Adult
- Human Lung Fibroblasts, Adult
- Human Retinal Muller Cells
- Human Articular Chondrocytes
- Human Retinal Pigment Epithelial Cells
- Human Pancreatic Islets of Langerhans Cells
- Human Kidney Podocyte Cells
- Human Renal Proximal Tubule Cells
Induced Pluripotent Stem Cells
Induced pluripotent stem cells (iPSCs) are pluripotent stem cells that have been artificially reprogrammed from many types of easily obtainable mature somatic cells such as cells from blood, skin, and urine samples. iPSCs have similar properties as those of embryonic stem cells (ESCs). Therefore, with their strong self-renewing and multidirectionally differentiative capacity, iPSCs can be differentiated into all types of functional cells of the body which are the basis for further clinical utilization [1].
These cells were firstly developed by Shinya Yamanaka’s laboratory in 2006 and 2007 from mouse [2] and human [3], respectively. As human iPSCs do not create the same ethical hurdles as human ESCs, the emergence of iPSCs has allowed researchers to experiment with stem cells from different backgrounds including both normal and diseased, thus revolutionizing pluripotent stem cell research in regenerative and personalized medicine, disease modelling, as well as drug screening.
Generation of iPSCs
With the continuous progress of science and technology, the iPSCs preparation process has become more and more mature, including several key steps, such as the selection of cell sources, the screening of reprogramming factors, and the selection of reprogramming vectors. Among them, the screening and integration techniques of transcription factors are the key aspect of reprogramming. More and more studies have shown that positive gene regulators, such as Oct3/4, Sox2 and Klf4, which maintain the pluripotency of ESCs, are necessary for reprogramming. Meanwhile, c-Myc plays an important role in the reprogramming mechanism and can improve editing efficiency. In addition, researchers have identified other transcription factors that can be used for reprogramming iPSCs, such as NANOG, LIN28, GLIS1, NR5A2 and SALL4. Different combinations of these factors besides OSKM (Oct3/4, Sox2, Klf4 and c-Myc), such as the combination of Oct3/4, Sox2, NANOG and LIN28, as well as the substitution of c-Myc and Oct3/4 by GLIS1 and NR5A2, especially with the addition of SALL4 which can significantly enhance the efficiency, have provided more choices for the preparation of iPSCs [4]. There are multiple ways to generate iPSCs with genetic reprogramming approach, including lentivirus or retrovirus-mediated gene transduction. iPSCs generation with genetic reprogramming approach is a slow process. It takes 3-4 weeks for human cells and 1-2 weeks for mouse cells.
Along with genetic reprogramming approach, chemical reprogramming methodology has also been developed. Recently, a highly efficient and reproducible chemical reprogramming method developed for the induction of human somatic cells to human iPSCs was published on Cell Stem Cell. Screened small molecule boosters together with a optimized chemical reprogramming condition enable a direct reprogramming process by promoting early stage cell proliferation and oxidative phosphorylation metabolic activities (Figure 1). Such discovery highlights a distinct chemical reprogramming pathway which can lead to a shortcut for the generation of human iPSCs through manipulation of cell fate transition [5].
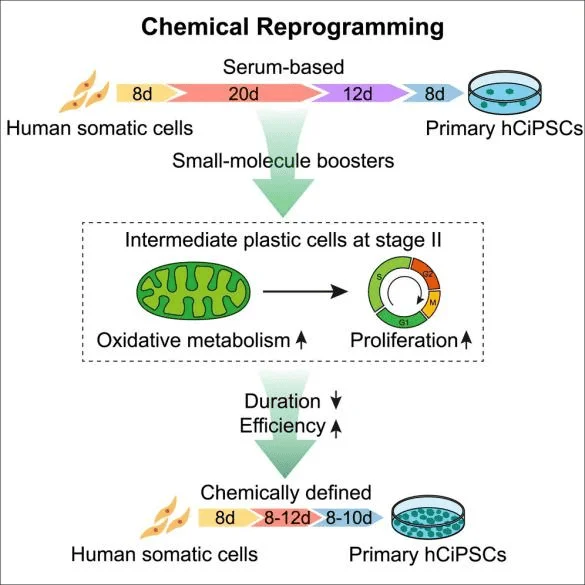
Figure 1. Highly efficient and rapid generation of human iPSCs by chemical reprogramming.
Advantages and Disadvantages of iPSCs
The advantages of iPSCs are mainly reflected in a few aspects: Firstly, compared to ESCs, iPSCs derived from somatic cells are compatible to non-invasive havesting and have solved the controversial issues over the destruction of embryos associated with ESCs use in research. Without ethical issues, researchers are likely to obtain more funding and support. Secondly, the characteristics of iPSCs are highly similar to those of human ESCs with equivalent stemness, which can be replicated indefinitely, and are suitable for large-scale culture. Moreover, iPSCs can be induced to differentiate into desired cell lines as demanded, and the characteristics are relatively stable from batch to batch, which can avoid the occurrence of inconsistent clinical efficacy. Furthermore, iPSCs can be generated from the somatic cells of patients with rare diseases (Figure 2) [6], these iPSCs would be further differentiated into different types of cells to produce disease affected cell populations, essentially producing a ‘disease in a dish’. After that, cells would be submitted to genome editing or drug screening, the former would lead to the generation of new cells that are genetically tailored to patients, therefore more effective for them to use. Meanwhile, this approach also helps eliminate the problem of immune system rejection.
Nevertheless, there are still disadvantages of iPSCs. For example, critical quality controls are necessary for clinical-grade iPSCs, strict quality attributes need to be established, an international standard shall be developed, and a global consensus should be built to facilitate the global application of iPSCs. Additionally, similar to ESCs, iPSCs administration also introduce the risk of tumorigenesis [7]. In terms of safety, reprogramming factors and the carriers should be carefully selected and precisely assessed. It is also necessary to clarify the biological mechanisms underlying tumorigenicity of iPSCs, especially the difference between teratoma formation of iPSCs and cancer initiation, contributing to an effective solution towards this critical issue for iPSC clinical applications. The good news for this industry is that low reprogramming efficiency of iPSC technology can now be changed by down-regulating the nucleosome remodeling and deacetylation. Apparently, progression in this area is still encouraging for the future of iPSCs and iPSC theraputics.
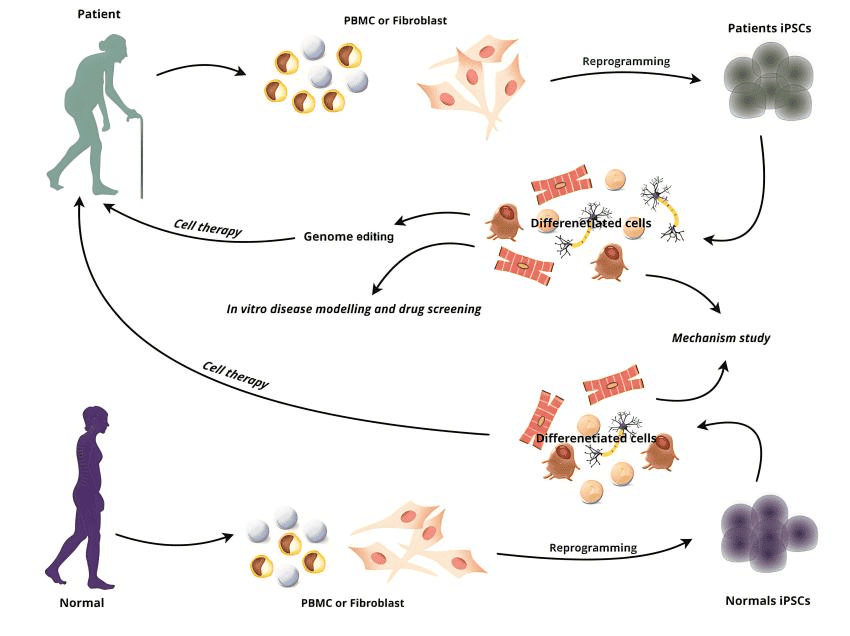
Figure 2. iPSCs is promising for cell therapy.
Research and Possible Applications of iPSCs
The iPSC research in ophthalmology is one of the pioneers. The first clinical study was initiated using autogenic iPSCs in human patients with age-related macular degeneration in 2013. After that, further advances using animal models have been made, and allogenic iPSC-derived retinal pigment epithelial (iPSC-RPE) cells have been used and reapplied to human patients. Most recently, the safety issues of transplantation with iPSC-RPE cells for age-related macular degeneration have been addressed to a certain extent [8]. Meanwhile, iPSCs have also been introduced in studies of cardiac disease modelling and drug testing [9]. Recently, iPSC-derived small extracellular vesicles have also been found to play a similar therapeutic role as iPSCs in myocardial injury treatment, but the limitation of cell therapy such as embolism and tumor occurrence can be avoided [10].
In addition, iPSCs have been used to model various neurological disorders including Alzheimer’s disease, Parkinson’s disease, neurodevelopmental disorders and neurodegenerative diseases [11-13]. Moreover, iPSC-derived organoids have been validated as promising research models to recapitulate human tissue damage and repair in vivo [14]. Human iPSCs have also been used to produce a variety of blood cells for the study of both hematologic and non-hematologic disorders [15]. Last but not least, the induction of iPSCs into pancreatic endocrine β-cells and immune cells could be the basis for creating novel disease models that can elucidate the disease mechanisms and facilitate the discovery of therapeutics drugs for diabetes [16] and cancer [17].
Aside from regenerative medicine, one more promising field of medicine, in which genetically patient specific iPSCs are useful, is gene therapy. So far, iPSCs have been used as a treatment method in proof-of-concept and preclinical studies of gene therapy for many life-threatening genetic diseases such as Sandhoff disease, severe combined immunodeficiency, severe congenital neutropenia, Hemophilia A, osteogenesis imperfecta, myotonic dystrophy and retinitis pigmentosa [7]. Although the methods of creating safe iPSCs for clinical use are still under development, iPSCs technology holds enormous potential especially in creating precise medical techniques such as creating models of genetic diseases and delivering therapeutic agents into patients via autotransplants.
Clinical Trials of iPSCs
As an emerging field of technology, iPSCs are developing rapidly. iPSC-related therapies have gradually become the focus of attention in pharmaceutical industry. To date, there are already 73 iPSC-related clinical trials listed on the website of ClinicalTrials.gov from around the world. Among them, there are 23 from United States, 13 from France, 7 from China, and 4 from Pakistan. Of all the diseases under current clinical studies, 17% is heart disease, including heart failure, coronary artery disease, congenital heart disease, inherited arrhythmias and valvulopathies, genetic and septic cardiomyopathy, etc. Genetic disease also takes part in 17% of the studies, covering Niemann Pick disease, imprinted disorders, Marfan’s syndrome, Morquio disease, Wilson disease, inborn errors of metabolism, and so on. Besides, 15% of conditions is neurological disease, 11% is retinal disease, and 8% is tumor, all of which are demonstrated in details in the statistical pie chart in Figure 3. Apparently, the top four diseases studied in clinical trails: heart disease, genetic disease, neurological disease and retinal disease are in parallel with the preclinical research information we have provided in the above section. Meanwhile, 7% of the clinical projects are just focusing on iPSCs manufacture and banking, indicating the great hopes scientists have for the future iPSC therapies. Nevertheless, the main research topics (93%) are unsurprisingly centered on the efficacy, safety and feasibility of the conducts of these trials.
In terms of cell products used as interventions, iPSCs are the predominant one, which accounts for 26% of the whole number. iPSC-derived cardiomyocytes, cardiomyocytes spheroids, cardiovascular progenitor cells and other cardiovascular cells, as well as 3D-engineered heart tissue derived from iPSCs together make up 22% of cell products used for various treatments in clinical trials. In addition, the proportion of the use of iPSC-derived RPE, neural retinal cells and other ocular cells derived from iPSCs is 12%. These top three cell types applied in clinical trails are also in high consistency with the research information we have summarized in the Research and possible Application of iPSCs section. Aside from these cells, iPSC-derived neural progenitor cells, dopaminergic neurons and other types of neurons, epithelial cells, endothelial cells, skeletal muscle progenitor cells, immune cells including NK cells, T cells and eosinophils, mesenchymal stem cells, hematopoietic stem cells, immune and brain organoids, as well as exosomes derived from iPSCs are also used as interventions.
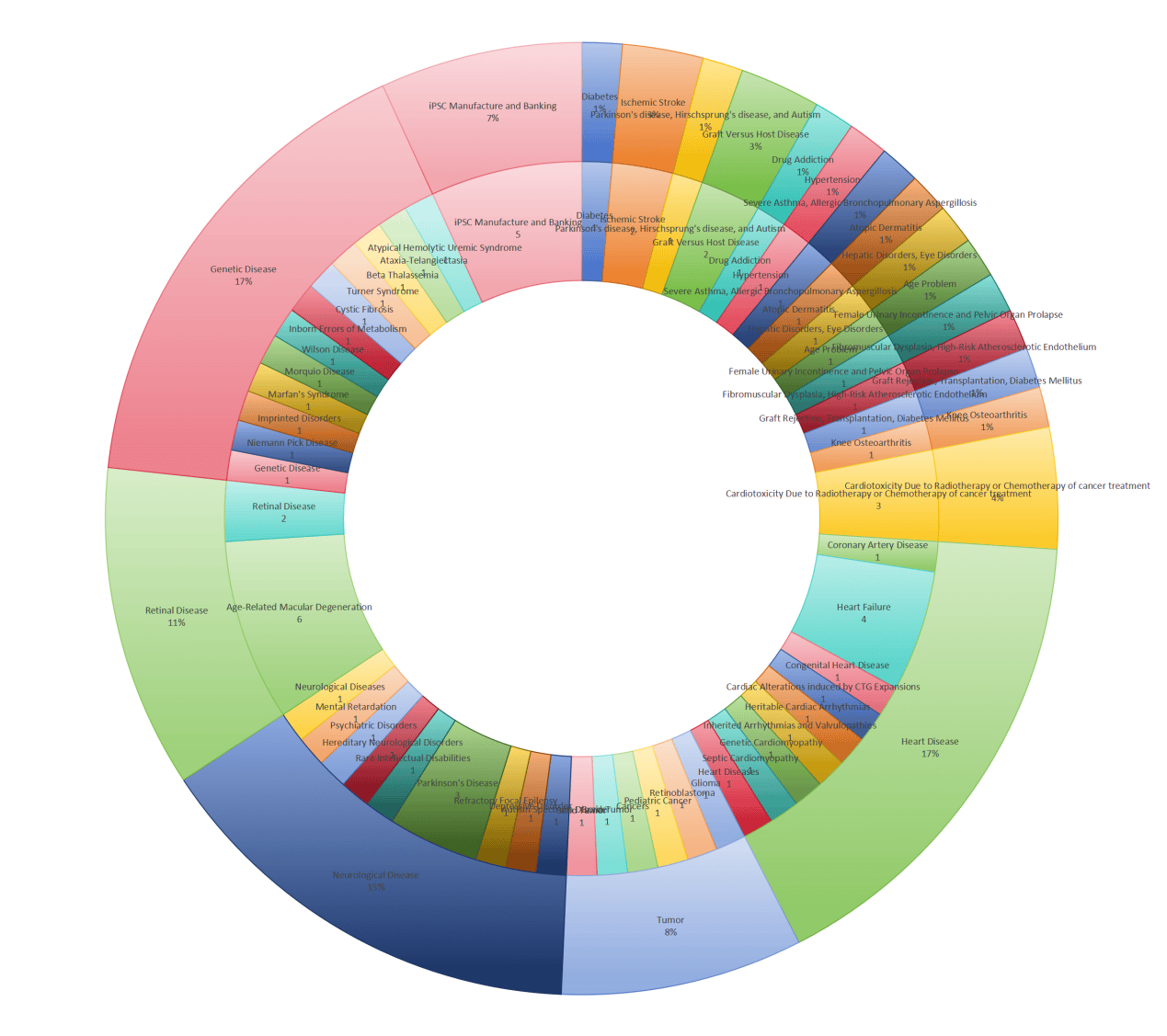
Figure 3. Statistical analysis of iPSC-related clinical trials listed on the website of ClinicalTrials.gov from around the world.
Conclusion
iPSCs are reprogrammed from mature somatic cells and have the capacity to differentiate into any type of body cells except for cells in extra-embryonic tissues such as the placenta. The discovery of iPSCs has made an invaluable contribution to stem cell biology, regenerative and personalized medicine, paving way for identifying the causes of refractory disease onsets and progressions to create and test new drugs and cell therapies. Although challenges still exist, iPSC technology may shed light on making real research and its process more concrete, simple, accessible and closer to society and eventually contributing to citizen science.
Reference
[1] Y.S. Chun, K. Byun, B. Lee, Induced pluripotent stem cells and personalized medicine: current progress and future perspectives, Anat Cell Biol 44(4) (2011) 245-55.
[2] K. Takahashi, S. Yamanaka, Induction of Pluripotent Stem Cells from Mouse Embryonic and Adult Fibroblast Cultures by Defined Factors, Cell 126(4) (2006) 663-676.
[3] K. Takahashi, K. Tanabe, M. Ohnuki, M. Narita, T. Ichisaka, K. Tomoda, S. Yamanaka, Induction of Pluripotent Stem Cells from Adult Human Fibroblasts by Defined Factors, Cell 131(5) (2007) 861-872.
[4] P. Karagiannis, K. Takahashi, M. Saito, Y. Yoshida, K. Okita, A. Watanabe, H. Inoue, J.K. Yamashita, M. Todani, M. Nakagawa, M. Osawa, Y. Yashiro, S. Yamanaka, K. Osafune, Induced Pluripotent Stem Cells and Their Use in Human Models of Disease and Development, Physiol Rev 99(1) (2019) 79-114.
[5] S. Liuyang, G. Wang, Y. Wang, H. He, Y. Lyu, L. Cheng, Z. Yang, J. Guan, Y. Fu, J. Zhu, X. Zhong, S. Sun, C. Li, J. Wang, H. Deng, Highly efficient and rapid generation of human pluripotent stem cells by chemical reprogramming, Cell Stem Cell 30(4) (2023) 450-459.e9.
[6] N. Yousefi, S. Abdollahii, M.A.J. Kouhbanani, A. Hassanzadeh, Induced pluripotent stem cells (iPSCs) as game‐changing tools in the treatment of neurodegenerative disease: Mirage or reality?, Journal of Cellular Physiology 235(12) (2020) 9166-9184.
[7] A. Fus-Kujawa, B. Mendrek, A. Trybus, K. Bajdak-Rusinek, K.L. Stepien, A.L. Sieron, Potential of Induced Pluripotent Stem Cells for Use in Gene Therapy: History, Molecular Bases, and Medical Perspectives, Biomolecules 11(5) (2021) 699.
[8] T. Maeda, M. Takahashi, iPSC-RPE in Retinal Degeneration: Recent Advancements and Future Perspectives, Cold Spring Harbor Perspectives in Medicine 13(8) (2023) a041308.
[9] L. Ye, X. Ni, Z.-A. Zhao, W. Lei, S. Hu, The Application of Induced Pluripotent Stem Cells in Cardiac Disease Modeling and Drug Testing, Journal of Cardiovascular Translational Research 11(5) (2018) 366-374.
[10] W.-T. Meng, H.-D. Guo, Small Extracellular Vesicles Derived from Induced Pluripotent Stem Cells in the Treatment of Myocardial Injury, International Journal of Molecular Sciences 24(5) (2023) 4577.
[11] M.A.M. Aboul-Soud, A.J. Alzahrani, A. Mahmoud, Induced Pluripotent Stem Cells (iPSCs)-Roles in Regenerative Therapies, Disease Modelling and Drug Screening, Cells 10(9) (2021) 2319.
[12] M. Sundberg, H. Bogetofte, T. Lawson, J. Jansson, G. Smith, A. Astradsson, M. Moore, T. Osborn, O. Cooper, R. Spealman, P. Hallett, O. Isacson, Improved Cell Therapy Protocols for Parkinson’s Disease Based on Differentiation Efficiency and Safety of hESC-, hiPSC-, and Non-Human Primate iPSC-Derived Dopaminergic Neurons, Stem Cells 31(8) (2013) 1548-1562.
[13] H. Wang, L.C. Doering, Induced Pluripotent Stem Cells to Model and Treat Neurogenetic Disorders, Neural Plasticity 2012 (2012) 1-15.
[14] M.F. Sobral-Reyes, D.R. Lemos, Recapitulating human tissue damage, repair, and fibrosis with human pluripotent stem cell-derived organoids, Stem Cells 38(3) (2020) 318-329.
[15] S.T.C. Christopher S Thom , Deborah L French, Mechanistic and Translational Advances Using iPSC-Derived Blood Cells, Journal of Experimental Pathology 1(2) (2020) 36-44.
[16] Y. Kondo, T. Toyoda, N. Inagaki, K. Osafune, iPSC technology‐based regenerative therapy for diabetes, Journal of Diabetes Investigation 9(2) (2018) 234-243.
[17] H. Kawamoto, K. Masuda, S. Nagano, Development of Immune Cell Therapy Using T Cells Generated from Pluripotent Stem Cells, Basic Immunology and Its Clinical Application, Springer Nature Singapore (2024) 207-217.
Copyright - Unless otherwise stated all contents of this website are AcceGen™ All Rights Reserved – Full details of the use of materials on this site please refer to AcceGen Editorial Policy – Guest Posts are welcome, by submitting a guest post to AcceGen you are agree to the AcceGen Guest Post Agreement – Any concerns please contact [email protected]